Autonomous Surface Vessels as Low-Cost TPOS Platforms for Observing the Planetary Boundary Layer and Surface Biogeochemistry
PIs: Meghan Cronin (NOAA PMEL), Dongxiao Zhang (UW CICOES), Adrienne Sutton (NOAA PMEL), Chris Meinig (NOAA PMEL)
Postdocs: Samantha Wills (UW CICOES), Jack Reeves Eyre (UW CICOES)
OCS Team Members: Nathan Anderson (UW CICOES), Patrick Berk (UW CICOES)
Description
Emerging technologies, such as Uncrewed Surface Vehicles (USV), hold great potential for providing important air-sea interaction observations without shiptime. Saildrone®1 USV (Figure 1), manufactured and piloted by Saildrone, Inc., are powered by wind and the Sun, and are capable of traveling ~16,000 nm, for up to 1-year. The sensor suite (Figure 2) includes a 3-dimensional sonic anemometer mounted atop the mast at 5m, and a suite of sensors that could be used to estimate air-sea heat, momentum and carbon dioxide fluxes, and potentially even direct covariance fluxes of momentum (i.e. wind stress) and buoyancy flux. This pilot study comprised three missions to the eastern and central tropical Pacific (Figure 3).
Mission 1 (Sep 1, 2017 – May 18, 2018) deployed 2 Gen4 drones enhanced with ASVCO2 systems. Launched from Alameda CA with a destination of 0, 125W, Mission 1 included a 3-week intercomparison against a WHOI surface flux mooring that was deployed at 10N, 125W as part of the NASA-funded Salinity Process in the Upper ocean Study-2 (SPURS-2). Mission 2 (Oct 3, 2018- February 2019) deployed 2 Gen4 and 2 Gen5 (enhanced with ASVCO2) drones and Mission 3 (June 8-Dec 8, 2019) deployed 4 modified Gen5 (2 enhanced with ASVCO2) drones. Both Mission 2 and 3 were launched from Hawaii and made observations to/from 0, 140W.
The objectives of this pilot study were to (1) test the performance of the Saildrone USV within the low-wind and high-current environment found in the tropical Pacific, (2) validate these surface observations in comparison to NDBC Tropical Pacific Observing System (TPOS) and NASA Salinity Process in the Upper ocean Study (SPURS)-2, and (3) to explore the spatial and temporal variability of air-sea interactions in the eastern and central tropical Pacific.
This pilot study was funded by the NOAA’s Global Ocean Monitoring and Observing Program (GOMO) as one of its six technology development projects and a NOAA contribution to TPOS2020. NOAA Office of Marine and Aviation Operations (OMAO) supported the 2018 and 2019 missions, and Saildrone, Inc. contributed two drones for each of these two missions. These supplements greatly expanded the scope of the original pilot study and are gratefully acknowledged.
1Saildrone is a trademark name; hereafter referred to without ®
Accomplishments
- Project year 1 (FY17) focused on developing a surface sensor suite similar to a TAO flux/CO2 moored buoy. New sensors for Saildrone included the ASVCO2 system, an ADCP, and solar and longwave radiometers.
- Project years 2-4 (FY18-FY20) saw three missions to the equatorial Pacific, with a total of 10 Saildrone USV that together travelled more than 65,000 nautical miles for 2150 cumulative seadays.
- All data are publicly available (see links below). In addition, winds, air temperature, relative humidity, barometric pressure, and sea surface temperature (SST) were made available in near-realtime to operational data centers through the Global Telecommunication Services
- Zhang et al. (2019) comparisons of saildrone and SPURS2 moored buoy data showed excellent agreement for all physical measurements, including for wind speed, sea surface temperature (SST), air-temperature, humidity, solar and long-wave radiation. The sea surface salinity (SSS) measurements by long term moorings tend to drift near the end of deployments due to biofouling; the newly deployed Saildrones provided useful records to correct these drifts.
- Sabine et al. (2020) showed that ASVCO2 systems are capable of long-term oceanic deployment and robust collection of air and seawater pCO2 within ± 2 µatm based on comparisons with established shipboard underway systems, with previously described Moored Autonomous pCO2 (MAPCO2) systems, and with companion ASVCO2 systems deployed side by side.
- Wills et al. (2021) showed that Saildrone USV can resolve atmospheric cold pools, identified as a 1.5C drop in 10 minutes. Other studies in progress show abrupt fronts on the northern edge of the equatorial cold tongue (Donohue et al.), the diurnal cycle in frontal zones (Cronin et al.), the influence of surface currents on air-sea fluxes (Zhang et al.), and direct covariance wind stress observations from Saildrone USV (Reeves Eyre et al.).
- After each mission, the Saildrone USV were re-engineered to reduce biofouling and aid navigation in swell and low-wind conditions. By Mission 3, the drones were able to perform repeat-squares on the equator. Through three 6-month field missions to the Tropical Pacific, this project has helped raise the NOAA technology Readiness Level (RL) of the Saildrone from RL 6, demonstration of prototype system in relevant environment, to RL 9, product deployed and used routinely within a research setting (NAO 216-105b).
Lessons Learned
- Saildrone USV provide high quality measurements for meteorological, oceanic (Zhang et al. 2019) & carbon parameters (Sabine et al. 2020), with excellent resolution of sharp fronts and rapid variability (Wills et al. 2021)
- Saildrone USV are not reliant on research vessels: deployed from shore, use wind and solar power, adaptive sampling, scalable
- Future central accessibility to remote parts of tropical Pacific: A Palmyra Atoll base (~162oW, 6oN) might provide efficient access to TPOS array
- Precise navigation is difficult in parts of the tropics (e.g. equatorial cold tongue) due to low winds and strong ocean currents. We don’t know how these USV will perform in other parts of the tropics, such as the western equatorial Pacific Warm Pool and its eastern edge, where the Trade winds are weak. More missions are needed to develop robust adaptive sampling strategies.
- Cost would need to be different for wide usage (process study vs. sustained obs costs).
- Saildrone USV ideally would be deployed as pairs or clusters to help distinguish temporal and spatial variability
- Saildrone USV could provide air-sea CO2 flux (and other) sections in regions not frequently visited by research vessels with underway CO2
- Saildrone USV could provide upper ocean current profiles from 6m to more than 60 m depth, in key regions, where the Equatorial Undercurrent rises interacts with the planetary oceanic boundary layer. It should be noted though that Saildrone USV are unable to provide upper ocean temperature profiles at this stage, and further work is needed to determine whether USV Saildrone ADCP measurements could be used to estimate equatorial upwelling.
Publications
Wills, S.M., M.F. Cronin, D. Zhang. 2021. Cold Pools Observed by Uncrewed Surface Vehicles in1the Central and Eastern Tropical Pacific. Submitted to Geophysical Research Letters
Sabine, C., A.J. Sutton, K. McCabe, et al. 2020. Evaluation of a new carbon dioxide system for autonomous surface vehicles, Journal of Atmospheric and Oceanic Technology 37(8): 1305-1317. https://doi.org/10.1175/JTECH-D-20-0010.1
Zhang, D., M.F. Cronin, C. Meinig, et al. 2019. Comparing air-sea flux measurements from a new unmanned surface vehicle and proven platforms during the SPURS-2 field campaign. Oceanography 32(2): 122-133. doi:10.5670/oceanog.2019.220.
Meinig, C., E.F. Burger, N. Cohen, et al. 2019. Public-private partnerships to advance regional ocean-observing capabilities: A Saildrone and NOAA-PMEL case study and future considerations to expand to global scale observing. Frontiers in Marine Science 6: 448. doi:10.3389/fmars.2019.00448.
Cronin, M.F., C.L. Gentemann, J. Edson, et al. 2019. Air-sea fluxes with a focus on heat and momentum. Frontiers in Marine Science 6: 430. https://doi.org/10.3389/fmars.2019.00430.
PMEL OCS / Saildrone Tech. Note 1-1: PMEL Science Requirements for ADCP on Saildrone.
PMEL OCS / Saildrone Tech. Note 2-1: Direct Flux Measurements on Saildrone.
Selected Media Links
- sciencemag.org/news/2018/03/fleet-sailboat-drones-could-monitor-climate-change-s-effect-oceans
- climate.gov/news-features/climate-tech/adaptable-and-driven-renewable-energy-saildrones-voyage-remote-waters
- popularmechanics.com/science/environment/a19376619/noaa-to-launch-a-navy-of-sailboat-drones-to-monitor-the-pacific-ocean/
- seattletimes.com/seattle-news/science/saildrones-go-where-humans-cant-or-dont-want-to-to-study-the-worlds-oceans
- https://www.saildrone.com/news/autonomous-vehicles-integral-tropical-pacific-observation
Data
The TPOS Saildrone data can be accessed via
- The Ocean Climate Station’s Saildrone data access webpage
- PMEL’s ERDDAP webpage
- Saildrone, Inc. data webpage
- Ocean Carbon Data System (OCADS)
For more information on the TPOS Saildrone missions in 2017, 2018, and 2019, see NOAA/PMEL’s Ocean Climate Stations webpage.
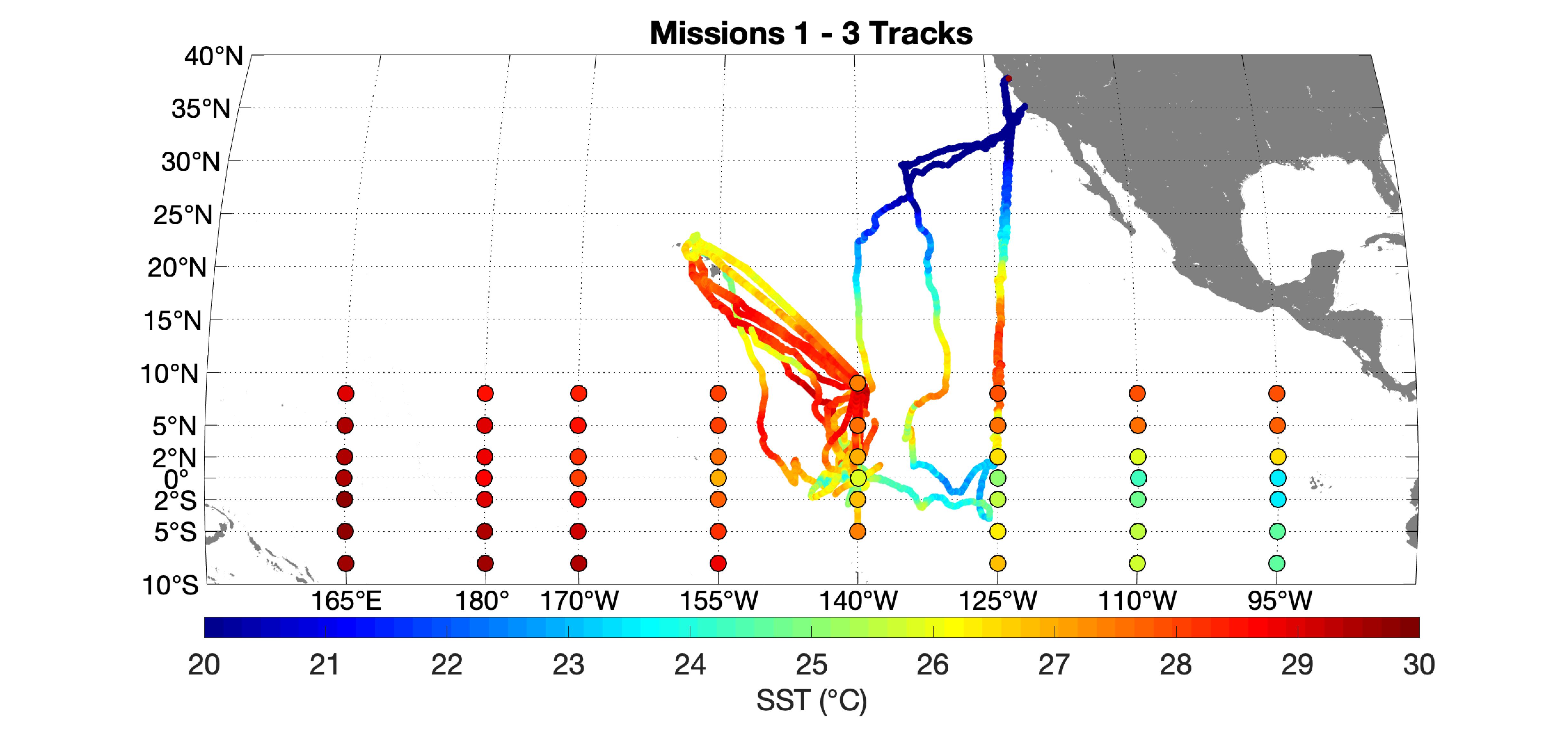
Figure 3. Missions 1 – 3 Saildrone tracks and TAO buoys throughout the tropical Pacific. The tracks are plotted as a function of 1-min mean SST, and the buoys are plotted as a function of their 2003 – 2019 climatological-mean SST.